APEEP electron precipitation models
and ionization data sets
The APEEP electron precipitation models and ionization data sets were developed by the University of Otago, the British Antarctic Survey, and the Finnish Meteorological Institute as a response to requests from the SOLARIS-HEPPA community. Atmospheric and climate simulations had been missing the ionization input from the so-called medium-energy electrons (MEE), with energies ranging between about 30 keV and 1 MeV.
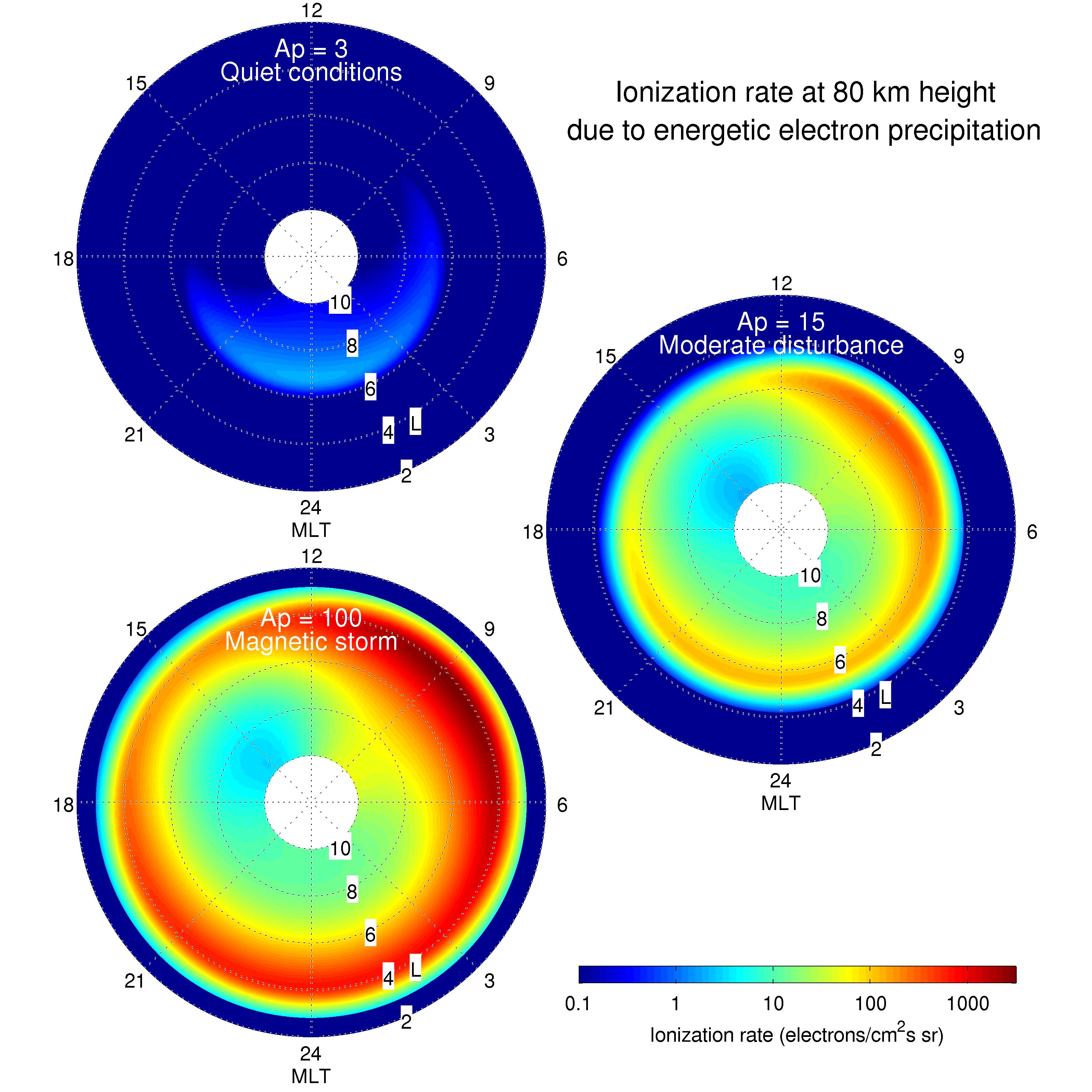
Figure: Ionization rates at 80 km altitude due to energetic electron precipitation according to the model of van de Kamp et al. (2018), as a function of MLT (Magnetic Local Time) and McIlwain L-shell value (a measure of magnetic latitude), for three levels of magnetic disturbance.
Using the electron flux observations of the MEPED instruments aboard the POES satellites, we have developed a series of precipitation models that provide atmospheric ionization rates for long-term simulations. The 0-degree channels of the SEM-2 MEPED package provides electron precipitation observations (see the background discussion in Rodger et al., 2010a; 2010b). In all cases low-energy proton contamination have been corrected for in the electron measurements following a validated algorithm (Whittaker et al., 2014), and observations during solar proton events have been suprpressed as these over-whelm the electron detectors. Due to the finding that the electron energy spectra of the precipitation fluxes can be well fitted by a power law (Whittaker et al., 2013), such fits have been applied to the MEPED flux measurements. The fitted energy-flux spectra (E = 30-1000 keV) have then been used to calculate atmospheric ionization rates. Methods vary slightly from data set to another, but mostly we have used the NRLMSISE-00 atmosphere (Picone et al., 2002) and parameterized ionization rate calculation by Fang et al. (2010).
The following provides a brief description of the available data sets, links to more detailed information, and access to the data sets. These data sets are freely available for scientific use. We ask you to cite the references, as given below for each data set, in any publication using the data.
PROXY MODELS, DATA SETS
APEEP v1, MEE precipitation model and ionization rates: years 1850-2015, temporal resolution 1 day, part of the CMIP6 recommendation. This is a statistical model fitted on zonally averaged MEPED data, it is described in detail by van de Kamp et al. (2016). This is a proxy model depending solely on the geomagnetic Ap index, which allows to extend the data set back in time. This model is recommended for the Coupled Model Intercomparison Project Phase 6 (CMIP6), see Matthes et al. (2017) for details. Access the data set through the SOLARIS-HEPPA CMIP6 page.
APEEP v2, MEE precipitation model and ionization rates: years 1932-2017, temporal resolution 1 day. This model is an improved version of APEEP v1. Noise-affected data were more carefully removed, to provide more realistic representations of low fluxes during geomagnetically quiet times. For a detailed description, see van de Kamp et al. (2018). Data access: contact Pekka Verronen (FMI).
APEEP-MLT v2, MEE precipitation model and ionization rates: years 1932-2017, temporal resolution 1 day (with 8 MLT sectors). Distinct from the earlier versions, which were zonally averaged, this model is dependent also on the magnetic local time (MLT) which is an important factor affecting precipitation flux characteristics. For a detailed description, see van de Kamp et al. (2018). Data access: contact Pekka Verronen (FMI).
DSTEEP v1, MEE precipitation model and ionization rates: years 1957-2015, temporal resolution 1 day. This is otherwise as APEEP v1, except that the Dst index is used as the proxy instead of Ap. See a detailed description in van de Kamp et al. (2016). Data access: contact Pekka Verronen (FMI).
DATA SETS CALCULATED DIRECTLY FROM MEPED DATA
ISSI MEE ionization rates: years 2000-2012, temporal resolution 3 hours. These rates are calculated directly from the zonally averaged electron fluxes observed by MEPED after some major corrections to the data. Note that compared to the other data sets, the energy range is different (50-2000 keV) and a different method was used to calculate ionization rates from spectra (Rees, 1989). For a detailed description, see Orsolini et al. (2018). Data access: contact Pekka Verronen (FMI).
NOHO MEE ionization rates: years 2013-2015, temporal resolution 3 hours. These rates are also calculated from MEPED observations. However, the flux data correction is different. For more information, see Newnham et al. (2018) and Orsolini et al. (2018). Data access: contact Pekka Verronen (FMI). Note: we do not recommend this data set for general use due to issues found in the flux data correction method.
REFERENCES:
Rodger, C. J., M. A. Clilverd, J. C. Green, and M. M. Lam (2010a), Use of POES SEM-2 observations to examine radiation belt dynamics and energetic electron precipitation into the atmosphere, J. Geophys. Res., 115, A04202, DOI:10.1029/2008JA014023
Rodger, C. J., B. R. Carson, S. A. Cummer, R. J. Gamble, M. A. Clilverd, J. C. Green, J.-A. Sauvaud, M. Parrot, and J. J. Berthelier (2010b), Contrasting the efficiency of radiation belt losses caused by ducted and nonducted whistler‐mode waves from ground-based transmitters, J. Geophys. Res., 115, A12208, DOI:10.1029/2010JA015880
Whittaker, I. C., R. J. Gamble, C. J. Rodger, M. A. Clilverd, and J.-A. Sauvaud (2013), Determining the spectra of radiation belt electron losses: Fitting DEMETER Instrument for Detecting Particle observations for typical and storm times, J. Geophys. Res. Space Physics, 118, DOI:10.1002/2013JA019228
Whittaker, I. C., C. J. Rodger, M. A. Clilverd, and J.-A. Sauvaud (2014), The effects and correction of the geometric factor for the POES/MEPED electron flux instrument using a multisatellite comparison, J. Geophys. Res. Space Physics, 119, 6386–6404, DOI:10.1002/2014JA020021
Fang, X., Randall, C. E., Lummerzheim, D., Wang, W., Lu, G., Solomon, S. C., and Frahm, R. A. (2010). Parameterization of monoenergetic electron impact ionization, Geophys. Res. Lett., 37, L22106. DOI:10.1029/2010GL045406
Picone, J. M., Hedin, A. E., Drob, D. P., and Aikin, A. C. (2002), NRLMSISE-00 empirical model of the atmosphere: Statistical comparisons and scientific issues, J. of Geophys. Res., 107(A12), 1468. DOI:10.1029/2002JA009430
van de Kamp, M., A. Seppälä, M.A. Clilverd, C.J. Rodger, P.T. Verronen, and I.C. Whittaker (2016), A model providing long-term datasets of energetic electron precipitation during geomagnetic storms, J. Geophys. Res. Atmos., 121, 12520-12540. DOI:10.1002/2015JD024212
Matthes, K., Funke, B., Andersson, M.E., Barnard, L., Beer, J., Charbonneau, P., Clilverd, M.A., Dudok de Wit, T., Haberreiter, M., Hendry, A., Jackman, C.H., Kretschmar, M., Kruschke, T., Kunze, M., Langematz, U., Marsh, D.R., Maycock, A., Misios, S., Rodger, C.J., Scaife, A., Seppälä, A., Shangguan, M., Sinnhuber, M., Tourpali, K., Usoskin, I., van de Kamp, M., Verronen, P.T. and Versick, S. (2017), Solar Forcing for CMIP6, Geosci. Model Dev., 10, 2247-2302. DOI:10.5194/gmd-10-2247-2017
van de Kamp, M., Rodger, C.J., Seppälä, A., Clilverd, M.A., and Verronen, P.T. (2018), An updated model providing long-term datasets of energetic electron precipitation, including zonal dependence, J. Geophys. Res. Atmos., 123, 9891-9915, DOI:10.1029/2017JD028253
Rees, M. H. (1989). Physics and chemistry of the upper atmosphere, Cambridge atmospheric and space science series. Cambridge, UK: Cambridge University Press. DOI:10.1017/CBO9780511573118
Orsolini, Y.J., C. Smith-Johnsen, D.R. Marsh, F. Stordal, C.J. Rodger, P.T. Verronen, and M.A. Clilverd (2018), Mesospheric nitric acid enhancements during energetic electron precipitation events simulated by WACCM-D, J. Geophys. Res. Atmos., 123, 6984-6998. DOI:10.1029/2017JD028211
Newnham, D.A., M.A. Clilverd, C.J. Rodger, K. Hendrickx, L. Megner, A.J. Kavanagh, A. Seppälä, P.T. Verronen, M.E. Andersson, D.R. Marsh, T. Kovacs, W. Feng, and J.M.C. Plane (2018), Observations and modelling of increased nitric oxide in the Antarctic polar middle atmosphere associated with geomagnetic storm driven energetic electron precipitation, J. Geophys. Res. Space, 123, 6009-6025. DOI:10.1029/2018JA025507